Large uncertainty exists in predictions of future transpiration due to complex interactions between plant physiology and the water and energy budgets.
The Science
Plant transpiration is the largest hydrologic flux of water globally, after precipitation, and therefore plays a large role in driving surface water availability. Transpiration responds to rising atmospheric CO2 at stomatal to whole-plant to regional-scales, with feedbacks between scales. This study reviewed the biophysical mechanisms by which rising CO2 impacts global scale plant transpiration. The authors then identify a path forward by which both empirical and modeling focused science approaches work together towards more mechanistic, evaluated prediction of transpiration under future conditions.
The Impact
Recent observations of rising plant transpiration at the global scale are consistent with increasing leaf area due to CO2 fertilization, but at odds with the well-known stomatal closure response. This increasing water demand results from a complex set of interacting factors and mechanisms, many of which are non-linear. This paper ultimately provides a testable framework of hypotheses regarding how transpiration responds globally to rising atmospheric CO2. The paper further serves as a call to arms for global empiricists and modelers to unify their efforts to better understanding and predicting transpiration under future conditions.
Summary
Here we review the myriad ways by which rising CO2 can directly and indirectly influence plant transpiration at the global scale. Many compensating mechanisms and feedbacks make the prediction of transpiration challenging with rising CO2. Global changes in plant transpiration in response to rising CO2 will manifest through droughts, vapor pressure deficit, plant physiological processes including shifting leaf area and phenology, and through forest loss (disturbance). We place these mechanisms into a testable framework of hypotheses that outlines a path forward for both empiricists and modelers. The impacts of changing transpiration at large scales are significant for water provision and utilization demands.
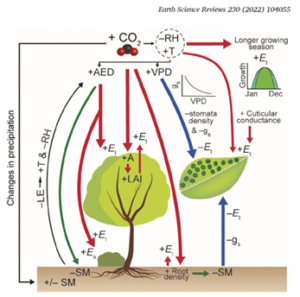
Contacts: Sergio Vicente-Serrano, Instituto Pirenaico de Ecología, svicen@ipe.csic.es
Brian Benscoter, U.S. Department of Energy, Biological and Environmental Research (SC-33), Environmental System Science, brian.benscoter@science.doe.gov
Funding
This work was supported by the Spanish Ministry of Science and FEDER; CROSSDRO project financed by the AXIS – sectorial climate impacts and pathways for sustainable transformation, JPI-Climate co-funded call of the European commission; NGM and LRL were supported by the Department of Energy’s Next Generation Ecosystem Experiment-Tropics. DGM acknowledges support from the European Research Council. AK acknowledges support from the European Union Horizon 2020 program.
Publication
Vicente-Serrano S, Miralles D, McDowell N, Brodribb T Domínguez-Castro F, Leung R, Koppa A. The uncertain role of rising atmospheric CO2 on global plant transpiration. Earth Science Reviews, p. 104055. https://doi.org/10.1016/j.earscirev.2022.104055